Pre-lab #4 The Celestial Globe
Over the course of a night, the constellations appear to move across the sky from east to west. However, ancient sky-watchers noted that the relative positions of stars remained unchanged as this nightly march took place. It was natural for those first astronomers to conclude that the stars were attached to a celestial sphere surrounding Earth—a canopy of stars like an astronomical painting on a heavenly ceiling. Figure P.2 shows how early astronomers pictured the stars as moving with this celestial sphere as it turned around a fixed, unmoving Earth. In reality stars appear to move in circles around a point in the sky very close to the star Polaris (better known as the Pole Star or the North Star). To the ancients, this point represented the axis around which the celestial sphere turned.
From our modern standpoint, the apparent motion of the stars is the result of the spin, or rotation, not of the celestial sphere but of Earth. Polaris indicates the direction—due north—in which the axis of Earth’s rotation points. Even though we now know that a revolving celestial sphere is an incorrect description of the heavens, we still use the idea as a convenient fiction that helps us visualize the positions of stars in the sky. The point where Earth’s axis intersects the celestial sphere in the Northern Hemisphere is known as the north celestial pole; it is directly above Earth’s North Pole. In the Southern Hemisphere, the extension of Earth’s axis in the opposite direction defines the south celestial pole. Midway between the north and south celestial poles lies the celestial equator, representing the intersection of Earth’s equatorial plane with the celestial sphere.
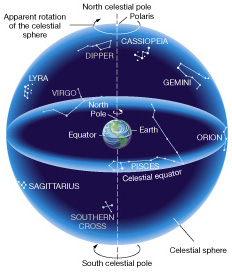
Figure P.2 The Celestial Sphere Planet Earth sits fixed at the hub of the celestial sphere, which contains all the stars. This is one of the simplest possible models of the universe, but it doesn’t agree with the facts that astronomers now know about the cosmos.
Celestial Coordinates
For more precise measurements, astronomers find it helpful to lay down a system of celestial coordinates on the sky. If we think of the stars as being attached to the celestial sphere centered on Earth, then the familiar system of latitude and longitude on Earth’s surface extends quite naturally to the sky. The celestial analogs of latitude and longitude are called declination and right ascension, respectively (Figure P.4). Just as latitude and longitude are tied to Earth, right ascension and declination are fixed on the celestial sphere. Although the stars appear to move across the sky because of Earth’s rotation, their celestial coordinates remain constant over the course of a night.
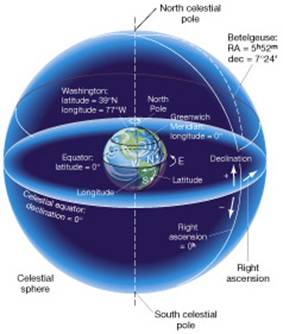
Declination (dec) is measured in degrees (°) north or south of the celestial equator, just as latitude is measured in degrees north or south of Earth’s equator. Thus, the celestial equator is at a declination of 0°, the north celestial pole is at +90° and the south celestial pole is at -90° (the minus sign here just means “south of the celestial equator”). Right ascension (RA) is measured in angular units called hours, minutes, and seconds, and it increases in the eastward direction. Like the choice of the Greenwich Meridian as the zero-point of longitude on Earth, the choice of zero right ascension is quite arbitrary—it is conventionally taken to be the position of the Sun in the sky at the instant of the vernal equinox (to be discussed in the next section).
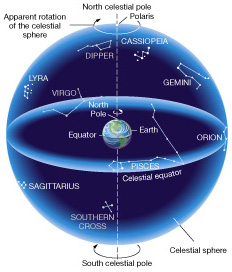
Because Earth revolves around the Sun, our planet’s darkened hemisphere faces in a slightly different direction each night. The change is only about 1° per night—too small to be easily discerned with the naked eye from one evening to the next. However, the change is clearly noticeable over the course of weeks and months. In six months Earth moves to the opposite side of its orbit, and we face an entirely different group of stars and constellations at night. Because of this motion, the Sun appears, to an observer on Earth, to move relative to the background stars over the course of a year. This apparent motion of the Sun on the sky traces out a path on the celestial sphere known as the ecliptic. The 12 constellations through which the Sun passes as it moves along the ecliptic—that is, the constellations we would see looking in the direction of the Sun if they weren’t overwhelmed by the Sun’s light—had special significance for astrologers of old. They are collectively known as the zodiac.
The ecliptic forms a great circle on the celestial sphere, inclined at an angle of about 23.5° to the celestial equator. In reality, as shown in the next Figure, the plane defined by the ecliptic is the plane of Earth’s orbit around the Sun. Its tilt is a consequence of the inclination of our planet’s rotation axis to its orbital plane.
The point on the ecliptic where the Sun is at its northernmost point above the celestial equator (see Figure) is known as the summer solstice (from the Latin words sol, meaning “sun,” and stare, “to stand”). It represents the point on Earth’s orbit where our planet’s North Pole is oriented closest to the Sun. This occurs on or near June 21—the exact date varies slightly from year to year because the actual length of a year is not a whole number of days. As Earth rotates on that date, points north of the equator spend the greatest fraction of their time in sunlight, so the summer solstice corresponds to the longest day of the year in Earth’s Northern Hemisphere and the shortest day in Earth’s Southern Hemisphere.
Six months later the Sun is at its southernmost point below the celestial equator—or, equivalently, the North Pole is oriented farthest from the Sun (see next Figure). We have reached the winter solstice (December 21), the shortest day in Earth’s Northern Hemisphere and the longest in the Southern Hemisphere.
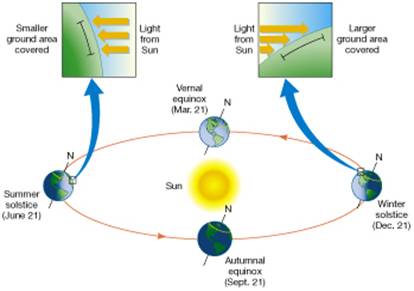
The Sun’s location with respect to the celestial equator and the length of the day combine to account for the seasons we experience. As illustrated, when the Sun is high in the sky, rays of sunlight striking Earth’s surface are more concentrated—spread out over a smaller area. As a result, the Sun feels hotter. Thus, in northern summer, when the Sun is highest above the horizon and the days are long, temperatures are generally much higher than in winter, when the Sun is low and the days are short.
The two points where the ecliptic intersects the celestial equator —that is, where Earth’s rotation axis is perpendicular to the line joining Earth to the Sun —are known as equinoxes. On those dates, day and night are of equal duration. (The word equinox derives from the Latin for “equal night.”) In the fall (in Earth’s northern hemisphere), as the Sun crosses from the northern into the southern celestial hemisphere, we have the autumnal equinox (on September 21). The vernal equinox occurs in spring, on or near March 21, as the Sun crosses the celestial equator moving north. The vernal equinox plays an important role in human timekeeping. The interval of time from one vernal equinox to the next—365.242 solar days—is known as one tropical year.
Contact Us
Physics and Astronomy Department
WPS 219
(615) 898-2130